The concept of the "crack evolution account" might sound complex, but when broken down, it provides a fascinating insight into the processes that shape geological formations and even influence technology development. This article aims to delve into the intricate world of crack evolution, exploring its significance in various fields such as geology, engineering, and material science. Unlocking the mysteries behind crack evolution not only enhances our understanding of natural processes but also aids in the development of safer and more efficient materials and structures. As we explore the crack evolution account, we'll dissect how cracks form, propagate, and ultimately impact the stability of structures, both natural and man-made. Understanding these processes is crucial for anyone involved in construction, manufacturing, or scientific research, as it enables the prediction and prevention of potential failures or disasters.
The journey into the realm of crack evolution is as much about history as it is about science. From the earliest human attempts to manipulate rocks and materials to the sophisticated engineering projects of today, crack evolution has played a pivotal role. By examining the past and present, this article will not only highlight the scientific principles at play but will also underscore the practical applications that have emerged as a result. Whether you're a student, a professional, or simply an enthusiast, this comprehensive exploration will provide valuable insights into the dynamic world of crack evolution.
This article is structured to cater to readers of various interests and expertise levels. We will begin with a foundational understanding of what crack evolution entails, followed by a detailed analysis of its mechanisms. Subsequently, we'll explore its applications across diverse industries, from construction to technology. The inclusion of frequently asked questions and a conclusion will ensure that all your queries are addressed, leaving you with a holistic understanding of the crack evolution account. So, let’s embark on this enlightening journey through the intricate processes and applications of crack evolution.
Table of Contents
- Biography of Crack Evolution
- Fundamentals of Crack Evolution
- Mechanisms of Crack Formation
- Crack Propagation and Its Implications
- Impact of Crack Evolution in Geology
- Engineering Applications of Crack Evolution
- Role in Material Science
- Ensuring Structural Integrity
- Impact on Technology Development
- Case Studies
- Future Trends in Crack Evolution
- Frequently Asked Questions
- Conclusion
Biography of Crack Evolution
Crack evolution, though a term primarily used in scientific and engineering contexts, has a rich history that parallels human civilization's progress. The understanding and manipulation of cracks date back to prehistoric times when early humans discovered that strategic cracking could aid in tool making. As societies evolved, so did their knowledge of how to control and utilize cracks for various purposes.
In ancient construction, crack evolution was both a challenge and a tool. The builders of the past, including those who erected the pyramids and aqueducts, had to contend with the natural tendency of materials to crack under stress. Their solutions laid the groundwork for modern engineering practices. Through trial and error, they developed methods to prevent unwanted cracks and harness them when necessary for structural advantages.
In the scientific realm, the study of crack evolution gained momentum during the industrial revolution. As machinery and infrastructure became more complex, so did the need for a deeper understanding of material behavior under stress. This period marked the beginning of the formal study of fracture mechanics, a field dedicated to understanding the formation, propagation, and impact of cracks in materials. The insights gained during this time have been instrumental in advancing both industrial and academic knowledge.
Today, crack evolution encompasses a broad range of studies and applications. It is a critical aspect of fields such as seismology, where understanding crack formation can help predict earthquakes, to aerospace engineering, where material integrity is paramount. The biography of crack evolution is thus a story of continuous learning and adaptation, illustrating humanity's quest to master the forces of nature and material science.
Fundamentals of Crack Evolution
To fully appreciate the complexity of crack evolution, it's essential to start with the basics. At its core, crack evolution refers to the process by which cracks form, grow, and eventually lead to the failure of a material or structure. This process is influenced by a multitude of factors, including the material's properties, the environmental conditions, and the type of stress applied.
Materials are inherently flawed, containing microscopic defects that can become sites for crack initiation. These defects, known as stress concentrators, amplify the stress experienced by the material, making it more susceptible to cracking. When a material is subjected to a force, these concentrators can cause localized stress, leading to the formation of a crack.
The type of stress applied can significantly influence crack evolution. Tensile stress, which stretches the material, is most likely to cause cracks, while compressive stress, which compresses the material, can sometimes close existing cracks. Shear stress, which involves opposing forces acting parallel to the material's surface, can also lead to crack formation, particularly in brittle materials.
Environmental factors such as temperature, humidity, and the presence of corrosive elements can further complicate crack evolution. High temperatures can weaken materials, making them more prone to cracking, while moisture can lead to corrosion, which exacerbates stress concentrators. Understanding these fundamentals is crucial for predicting and managing crack evolution in various applications.
Mechanisms of Crack Formation
Crack formation is a complex process that involves multiple mechanisms, each contributing to the overall evolution of the crack. One primary mechanism is mechanical loading, where external forces induce stress within the material, leading to crack initiation. These forces can be static, such as the weight of a structure, or dynamic, like the vibrations caused by machinery or seismic activity.
Another significant mechanism is thermal fatigue, which occurs when a material experiences repeated cycles of heating and cooling. This constant expansion and contraction create stress within the material, eventually leading to crack formation. Thermal fatigue is a common challenge in industries such as aerospace and automotive, where materials are exposed to extreme temperature fluctuations.
Corrosion fatigue is a mechanism that combines mechanical stress and chemical degradation. In environments where corrosive agents are present, such as saltwater or industrial pollutants, materials can weaken over time. The combination of corrosion and stress accelerates crack formation, posing a significant risk to the integrity of structures and machinery.
Hydrogen embrittlement is another mechanism that can lead to crack formation. This occurs when hydrogen atoms diffuse into a material, causing it to become brittle and fracture under stress. This phenomenon is particularly concerning in high-strength steels and other alloys used in critical applications, such as pipelines and pressure vessels.
Crack Propagation and Its Implications
Once a crack has formed, its propagation—the process by which it grows and extends—is a critical aspect of crack evolution. Crack propagation can lead to catastrophic failure if not properly managed, making understanding this process vital for maintaining structural integrity.
The rate of crack propagation is influenced by several factors, including the material's toughness, the stress intensity, and the presence of external factors such as corrosion or temperature changes. Materials with high toughness can absorb more energy before fracturing, slowing down crack propagation. Conversely, brittle materials tend to propagate cracks more rapidly.
Stress intensity, a measure of the stress concentration at the crack tip, plays a crucial role in crack propagation. When the stress intensity exceeds a material's fracture toughness, the crack will grow. Engineers use this knowledge to design structures that minimize stress concentrations and prevent crack propagation.
Crack propagation can occur in different modes, depending on the direction and type of applied stress. Mode I, or opening mode, involves tensile stress perpendicular to the crack plane. Mode II, or sliding mode, involves shear stress parallel to the crack plane. Mode III, or tearing mode, involves out-of-plane shear stress. Each mode has unique characteristics and requires specific strategies for management and prevention.
Impact of Crack Evolution in Geology
In the field of geology, crack evolution plays a pivotal role in shaping the Earth's landscape. Geological processes such as tectonic movements, volcanic activity, and erosion are all influenced by the formation and propagation of cracks within the Earth's crust.
One of the most significant impacts of crack evolution in geology is the formation of faults and fractures. These features result from the movement of tectonic plates and can lead to earthquakes when stress is released suddenly. Understanding the mechanisms of crack evolution in geological contexts is crucial for predicting seismic activity and mitigating its impact on human populations.
Crack evolution also influences volcanic activity. As magma rises through the Earth's crust, it creates pressure that can lead to the formation of cracks. These cracks serve as pathways for magma to reach the surface, resulting in volcanic eruptions. Monitoring crack evolution in volcanic regions helps in predicting eruptions and implementing safety measures.
Erosion, another geological process, is closely linked to crack evolution. Water, wind, and ice can exploit existing cracks in rocks, gradually breaking them down over time. This process is essential for the formation of landscapes such as valleys, canyons, and cliffs. By studying crack evolution, geologists can better understand the forces shaping the Earth's surface.
Engineering Applications of Crack Evolution
In engineering, understanding crack evolution is essential for designing safe and reliable structures. Engineers use principles of fracture mechanics to predict and prevent crack formation and propagation in materials ranging from concrete to metals and composites.
One application of crack evolution in engineering is in the design of bridges and buildings. Engineers must consider the potential for cracks to form due to factors such as load, temperature changes, and environmental exposure. By understanding crack evolution, they can design structures that distribute stress evenly, reducing the risk of failure.
In the aerospace industry, crack evolution is a critical consideration in the design and maintenance of aircraft. The materials used in aircraft must withstand extreme stress and temperature fluctuations, making them susceptible to crack formation. Engineers use non-destructive testing techniques to monitor for cracks and ensure the safety of aircraft.
Automotive engineers also rely on an understanding of crack evolution to design vehicles that are both safe and durable. From the body structure to the engine components, every part of a car is subject to stress that can lead to crack formation. By studying crack evolution, engineers can develop materials and designs that enhance the longevity and safety of vehicles.
Role in Material Science
Material science is deeply intertwined with the study of crack evolution. The development of new materials with enhanced properties often hinges on understanding and controlling crack formation and propagation.
Researchers in material science focus on creating materials that are both strong and tough, able to resist crack initiation and slow crack propagation. This involves manipulating the microstructure of materials to enhance their resistance to stress and environmental factors.
Advanced materials such as composites and alloys are designed with crack evolution in mind. These materials are engineered to have a high fracture toughness, making them ideal for applications where reliability and safety are paramount. For instance, in the aerospace and automotive industries, lightweight composites are used to reduce weight while maintaining strength and durability.
Nanotechnology is another area where crack evolution plays a crucial role. By understanding how cracks form and propagate at the nanoscale, scientists can develop materials with unprecedented properties. These materials have the potential to revolutionize industries ranging from electronics to medicine.
Ensuring Structural Integrity
Ensuring the structural integrity of buildings, bridges, and other infrastructure is a primary concern for engineers, and understanding crack evolution is key to achieving this goal. Structural integrity refers to a structure's ability to withstand its intended load without experiencing failure, which often results from crack formation and propagation.
Regular inspection and maintenance are essential components of ensuring structural integrity. Engineers use various techniques, such as ultrasonic testing and radiography, to detect cracks before they lead to failure. By monitoring crack evolution, engineers can identify areas that require reinforcement or repair.
Design strategies also play a significant role in maintaining structural integrity. By incorporating redundancy into a structure, engineers can ensure that if one component fails, others can take on the load, preventing catastrophic collapse. Additionally, the use of materials with high fracture toughness can reduce the risk of crack propagation.
In earthquake-prone regions, understanding crack evolution is vital for designing structures that can withstand seismic activity. Engineers use seismic design principles to create buildings and bridges that can absorb and dissipate energy, reducing the likelihood of crack formation and structural failure during an earthquake.
Impact on Technology Development
The study of crack evolution has significant implications for technology development, influencing everything from the microchips in our devices to the development of new energy sources.
In the field of electronics, understanding crack evolution is crucial for developing reliable microchips and circuit boards. These components are subject to stress from thermal cycling and mechanical forces, making them susceptible to crack formation. By understanding crack evolution, engineers can design more durable electronic components, enhancing the performance and lifespan of devices.
The energy sector also benefits from insights into crack evolution. In the development of renewable energy technologies, such as wind turbines and solar panels, understanding how cracks form and propagate can lead to more efficient and reliable energy sources. For instance, improving the durability of turbine blades and solar cells can increase their lifespan and reduce maintenance costs.
Furthermore, the exploration of new materials for energy storage, such as batteries and fuel cells, relies on understanding crack evolution. Ensuring that these materials can withstand repeated charging and discharging cycles without cracking is essential for developing efficient and long-lasting energy storage solutions.
Case Studies
To illustrate the practical applications of crack evolution, let's explore a few case studies across different industries.
In the construction industry, the Millennium Bridge in London serves as a case study in managing crack evolution. During its initial opening, the bridge experienced excessive swaying due to unexpected pedestrian-induced vibrations. Engineers analyzed the crack evolution in the bridge's components and implemented dampers to reduce stress and prevent crack formation, ensuring the bridge's stability.
In the aerospace sector, the Aloha Airlines Flight 243 incident highlights the importance of crack evolution understanding. A section of the aircraft's fuselage peeled away during flight due to undetected fatigue cracks. This incident prompted a reevaluation of inspection procedures and materials used in aircraft, leading to improved safety standards.
The automotive industry also provides a compelling case study in the form of the Ford Pinto. In the 1970s, the Pinto's fuel tank design made it susceptible to rupture and fire in rear-end collisions. Understanding the crack evolution in the tank's structure led to design changes that improved safety and reduced the risk of failure.
Future Trends in Crack Evolution
Looking ahead, the study of crack evolution is poised to continue influencing various fields, driven by technological advancements and societal needs.
In the realm of materials science, the development of smart materials that can detect and repair cracks autonomously is a promising trend. These materials could revolutionize industries ranging from aerospace to civil engineering by increasing the lifespan and safety of structures.
Advancements in computational modeling and simulation are also shaping the future of crack evolution studies. These tools allow engineers and scientists to predict crack formation and propagation with greater accuracy, leading to more efficient designs and preventive measures.
The integration of artificial intelligence and machine learning in crack evolution research offers exciting possibilities. By analyzing vast amounts of data, these technologies can identify patterns and predict crack behavior, enhancing our ability to manage and prevent failures.
Frequently Asked Questions
What is crack evolution?
Crack evolution refers to the process by which cracks form, grow, and potentially lead to the failure of a material or structure. It involves understanding the mechanisms of crack initiation and propagation under various conditions.
Why is understanding crack evolution important?
Understanding crack evolution is crucial for designing safe and reliable structures, preventing material failure, and enhancing the durability of engineering components. It is essential in fields such as construction, aerospace, and material science.
How do environmental factors affect crack evolution?
Environmental factors such as temperature, humidity, and corrosive elements can significantly impact crack evolution. High temperatures can weaken materials, moisture can lead to corrosion, and corrosive environments can accelerate crack formation and propagation.
What are the different modes of crack propagation?
Crack propagation can occur in three primary modes: Mode I (opening mode), where tensile stress acts perpendicular to the crack plane; Mode II (sliding mode), where shear stress acts parallel to the crack plane; and Mode III (tearing mode), where out-of-plane shear stress is involved.
Can cracks be repaired once they form?
Yes, cracks can often be repaired using various techniques, depending on the material and the extent of the damage. Common methods include welding, adhesive bonding, and using sealants or fillers. The goal is to restore the material's integrity and prevent further crack propagation.
What role does crack evolution play in earthquake prediction?
In seismology, understanding crack evolution is crucial for predicting earthquakes. The formation and propagation of cracks within the Earth's crust can indicate tectonic activity, helping scientists identify potential earthquake zones and assess risks.
Conclusion
The exploration of the crack evolution account takes us through a journey of scientific principles, historical insights, and practical applications. From the challenges faced by ancient builders to the sophisticated analysis in modern engineering, understanding crack evolution remains a cornerstone of material science and structural integrity. By mastering this complex process, we continue to build safer, more reliable, and more durable structures, paving the way for a future where technology and nature coexist harmoniously.
As our understanding of crack evolution deepens, the potential for innovation grows. With advances in materials science, computational modeling, and artificial intelligence, the future holds exciting possibilities for managing and harnessing the power of cracks. By staying informed and embracing these developments, we can ensure that our structures and technologies remain resilient in the face of evolving challenges.
In conclusion, the study of crack evolution is an ever-evolving field, vital for the advancement of technology and the safety of our built environment. By continuing to explore and understand this intricate process, we can unlock new potential in the materials and systems that shape our world.

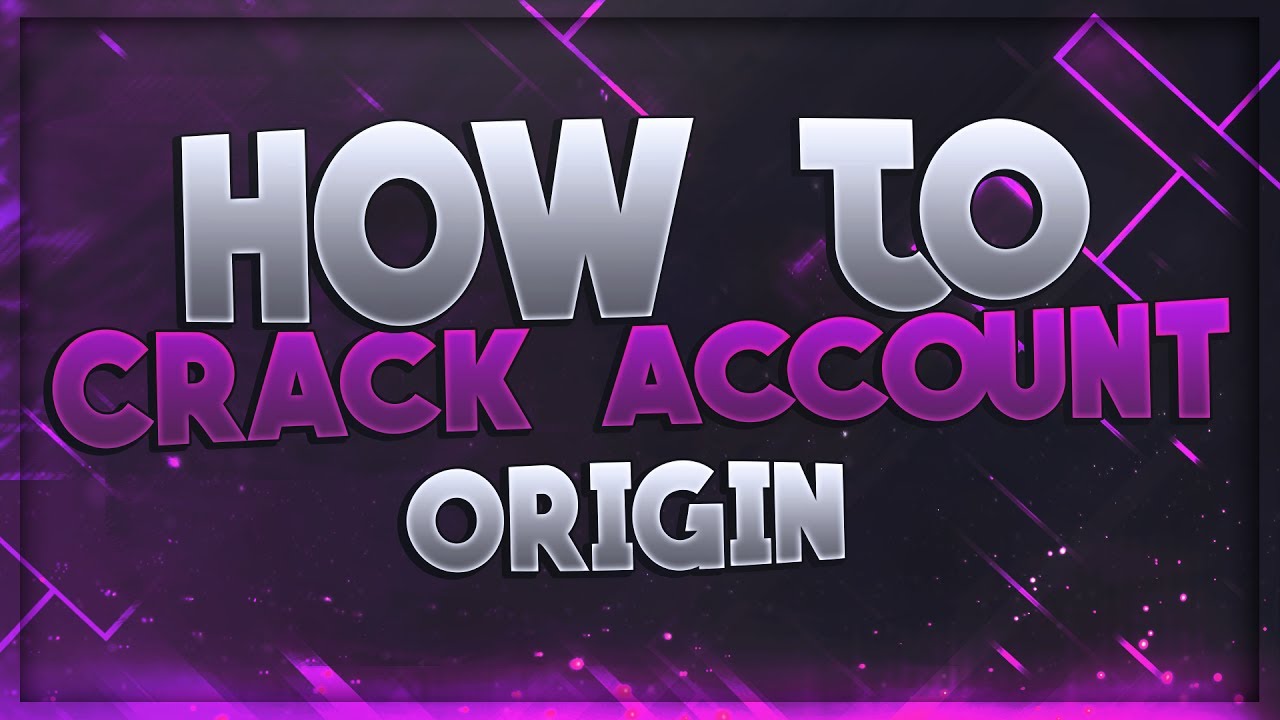